A New Method for the Early Detection of Edible Oil Oxidation for Packaging Optimization

Content originally authored by Vaughan Langford, Syft Technologies. Quantum Analytics is an authorized distributor for Syft Technologies in the United States.
Edible oils are used in a wide range of culinary applications. Oils containing unsaturated fatty acids have been demonstrated to offer health benefits over their saturated counterparts but are unfortunately significantly susceptible to oxidation. This oxidation is a significant cause of reduced shelf-life in these oils and can be a good indicator of the efficacy of their packaging.
In this white paper we briefly reviewed the chemistry of edible oils and their oxidation. Then we describe how oxidation can be detected early and with high specificity in order to diagnose leaks and inefficiencies in the packaging, using Selected Ion Flow Tube Mass Spectrometry (SIFT-MS).
Introduction
Edible oils are composed of fatty acids (high molecular weight carboxylic acids) and glycerol. Each glycerol molecule is bonded to three fatty acids via ester linkages, forming triglycerides (triacylglycerols), as shown in Figure 1.
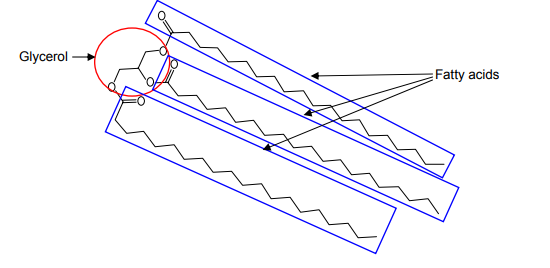
Figure 1. A triglyceride composed of glycerol and three fatty acids. Here three eicosanoic acid (arachidic acid) units, each having 20 carbon atoms, are shown. This acid is found in peanut oil, for example.
Vegetable oils normally contain fatty acids between 12 and 26 carbons in length. A fatty acid with only single carbon-carbon bonds (as shown in Figure 1) is a saturated fatty acid because the carbons are bonded to the maximum number of hydrogen atoms. Fatty acids that contain carbon-carbon double bonds are described as unsaturated because more hydrogen atoms could be added to them (this is sometimes done industrially to create so-called hydrogenated vegetable oils from unsaturated oils).
The presence of a double bond in the fatty acid significantly changes its chemical behavior. In particular, increasing the number of double bonds causes the rate of oxidation of the fatty acid to increase. This is illustrated in Table 1, which lists some of the commercially significant fatty acids found in vegetable oils. When fatty acids oxidize, the oxidation products adversely affect the taste and smell of the oil.
However, fatty acids with multiple double bonds (“polyunsaturated” fatty acids) have a beneficial effect on health, helping to protect against heart disease when consumed.1 So the most desirable situation is to use oils which have a high percentage of unsaturated fatty acids, but to prevent oxidation as much as possible.
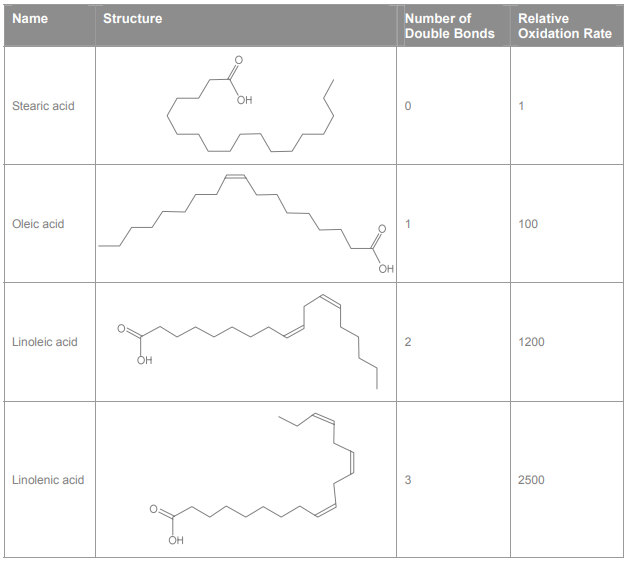
Table 1. Structures and relative oxidation rates (25 °C) of several important fatty acids found in vegetable oils.2
Oxidation of Edible Oils
Oil oxidation is a chain reaction involving free radicals. It starts when the ever-present radical, oxygen, is excited (often catalytically by another species) and becomes more reactive. Oil oxidation is more readily initiated under high light levels and all reactions proceed faster at elevated temperatures. Oil oxidation occurs in two stages called primary and secondary oxidation.
Primary oxidation consists of oxygen adding to fatty acids at the double bond position to form peroxides. This is more likely to occur to fatty acids with more double bonds. The peroxides have no taste or smell, so are very insidious. An oil can have a large percentage of peroxides (measured in milliequivalents of oxygen per kilogram, or meqO2/kg) but still smell and taste the same as when it was fresh. The change to the oil’s sensory attributes occurs when the peroxides break down in the process of secondary oxidation.
Secondary oxidation produces volatile compounds, which affect the taste and smell of the oil. Table 2 shows the two most abundant volatile oxidation products for three important fatty acids. Each fatty acid yields quite different volatile oxidation products, all of which differ from natural, desirable volatile compounds in the oils. These compounds give oxidized oil its distinctive aroma, which trained oil tasters describe as “rancid”, but it can also be likened to the smell of varnish.
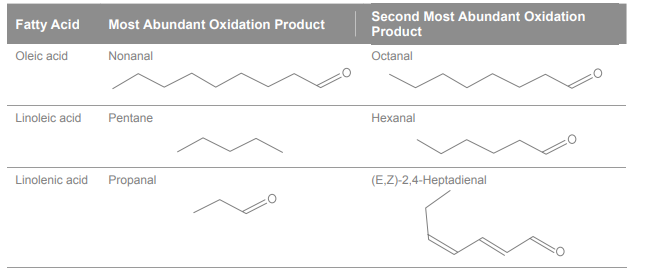
Table 2. Three fatty acids commonly found in vegetable oils and their first and second highest concentration volatile secondary oxidation products.
Unfortunately a direct correlation does not exist between the proportions of fatty acids in the oil and the proportions of volatile oxidation products in the headspace. However, the degree of oxidation can be determined by comparing the volatile profile of the test oil with reference standards. This is the method used in sensory analysis.
Refined Oils
For many oils (e.g. fish, canola, sunflower and peanut oil) the volatile oxidation products constitute the entire oil aroma because the refining process removes all original volatile aroma compounds from the oil. Hence oxidation can quickly produce a noticeable, undesirable change in the flavor of these oils.
Refined oils are more susceptible to oxidation because refining removes their natural antioxidants. Antioxidants scavenge radicals, preventing them from oxidizing the fatty acids. Vitamin E (tocopherol), a naturally occurring antioxidant, is often added after refining to slow the oxidation process.
Unrefined Oils
For oils that are marketed in an unrefined state (e.g. olive and sesame oil), it is more difficult to determine the extent of oxidation. These oils contain other volatile compounds that give them their characteristic, desirable aromas. Oxidation does, however, produce new compounds with undesirable aromas that gradually overwhelm the desirable aroma of the fresh oil
Unrefined oils retain their natural antioxidants and hence do not usually have antioxidants added to them.
Traditional Methods for Detection of Oxidation in Edible Oils
At present an oil’s oxidative state is normally only measured during or immediately after the manufacturing process. After manufacture it is commonly assumed that an oil will be appropriately handled and stored so that it maintains an acceptable quality until its ‘use by’ date.
Current prescribed tests to measure olive oil oxidation are the peroxide value test and the sensory test. Peroxide value is a measure of initial fatty acid breakdown products, and is assessed via a titration method. Although it is a rapid and reliable test, it must be performed by trained personnel in a laboratory setting. Skilled sample preparation is required to generate the necessary reactive species and reference standards are needed to calibrate results.
The sensory test measures VOCs and some non-volatile compounds, and relies on the human senses of taste and smell to make an assessment. A trained panel conducts this test under strictly controlled conditions, essentially duplicating the end user’s evaluation. The primary drawback of this test is its time-consuming nature, as conditions have to be carefully controlled and a maximum of three oils can be tested per day by each sensory panel.
Several other analysis methods exist for measuring oil oxidation, all based on measuring either peroxides or VOCs. Most measure the occurrence of a particular type of functional group, such as the anisidine and heptanal value tests.3
SIFT-MS and Its Suitability for Detection of Oil Oxidation
SIFT-MS is a powerful analytical technique that uses chemical ionization reactions coupled with mass spectrometric detection to rapidly quantify targeted VOCs. VOCs are identified and quantified in real time from whole-gas samples, based on the known rate coefficients for reaction of the chemically ionizing species (so-called reagent ions) with the target analytes.
The reagent ions used in this case are H3O+, NO+ and O2+, which react with trace VOCs in well characterized ways but do not react with the major components of air. Generally the soft chemical ionization used in SIFT-MS yields a smaller range of product ions than is common in electron impact mass spectrometry (as used by gas chromatography mass spectrometry (GC-MS), for example). Hence the need for gas chromatographic separation of the sample is circumvented, speeding sample throughput and providing instantaneous quantification of VOCs. Use of several reagent ions to independently quantify target analytes also greatly reduces interferences, markedly increasing the specificity of SIFT-MS versus competing whole-gas analysis technologies.
These characteristics make SIFT-MS ideally suited to rapid, sensitive detection and quantification of volatile secondary oxidation products arising from edible oils (such as those shown in Table 2). Moreover, the selectivity of SIFT-MS enables it to readily distinguish the volatile oxidation products from the favorable aroma compounds in unrefined oils. Several examples of the application of SIFT- MS to detection of edible oil oxidation are given below.
Olive Oil
Olive oil, like all edible oils, begins to oxidize immediately after production. It is an unrefined oil with a complex green, pungent flavor when fresh. When rancidity has reached a certain point, the rancid flavors overwhelm the favorable flavors. Figure 2 shows the results of an accelerated oxidation experiment on a fresh oil (using elevated temperature of 60 °C). These results demonstrate the ability of SIFT-MS to detect the degree of oxidation in the presence of favorable aroma compounds, such as trans-2-hexenal.
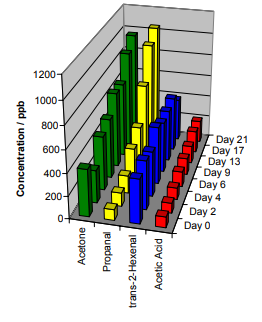
Figure 2. Oxidation of olive oil at 60 °C over a three-week period. The three most prominent oxidation products are shown along with trans-2-hexenal, an important flavor compound that did not change in concentration.
Soybean oil and mayonnaise
Soybean oil is a refined vegetable oil that, among other uses, is commonly employed as the base for mayonnaise. In Figure 3, results from oxidation of generic soybean oil (Malaysian origin) and a leading brand of mayonnaise (North American origin) are shown. Both products were protected with antioxidant and hence oxidation has taken some weeks at room temperature even though only 11 grams of sample was used and exposed to one liter of laboratory air.
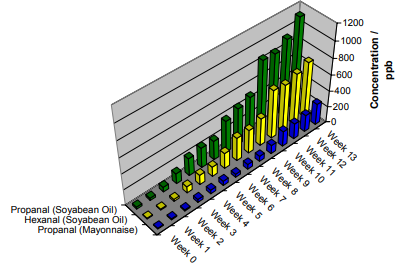
Figure 3. Detection of oxidation in 11-gram samples of soybean oil and mayonnaise at room temperature over a three-month period, using SIFT-MS.
Fish oil
As a rich source of omega-3 unsaturated fatty acids, consumption of fish oil provides a range of health benefits. However, fish oil is very sensitive to oxidation, which leads to rancidity. Figure 4 shows the most abundant VOCs detected during the room-temperature oxidation of approximately 5 ml of fish oil (from 1-ml capsules of USA origin) in a one-liter glass jar. The fish oil was observed to oxidize very rapidly.
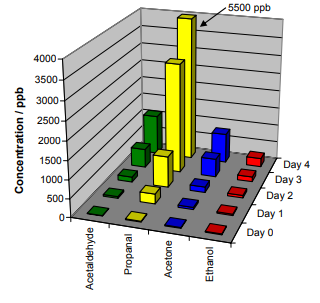
Figure 4. Dominant compounds detected and quantified, using SIFT-MS, as fish oil oxidized. The NO+ reagent ion consistently provided the best quantification.
Summary
Edible oil oxidation is a complicated radical chain reaction where oxygen breaks down fatty acids to form volatile compounds. The rate of oxidation is affected by heat, light, the fatty acid profile and the content of antioxidants in the oil. The progress of oil oxidation can be assessed by the measurement of peroxides and volatile compounds. SIFT-MS provides very sensitive, rapid and specific detection of the volatile oxidation products.
Further information on SIFT-MS, including background, technical and application specific information, is available on the Quantum Analytics website www.lqa.com/products/sift-ms/
Alternatively, questions and inquiries can be sent directly to Daniel Brown via email at dbrown@lqa.com.
References
1. V. Wijendran, K.C. Hayes (2004). “Dietary n-6 and n-3 Fatty Acid Balance and Cardiovascular Health”, Annu. Rev. Nutr., 24, pp. 597-615.
2. H.-D. Belitz, W. Grosch, P. Schieberle, P. (2004). Food Chemistry, 3rd Ed., Springer, Germany, pp. 190-201.
3. H.-D. Belitz, W. Grosch, P. Schieberle, P. (2004). Food Chemistry, 3rd Ed., Springer, Germany, pp. 670-671.
To learn more about the author, Daniel Brown you can visit his contributor page or email him directly at dbrown@lqa.com